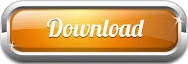

It can also induce silk fibroin–hyaluronic acid to generate hydrogel by ultrasound. When mixed with hydroxyapatite, the SF membrane can enhance mechanical properties and promote cell proliferation and has applications in wound healing or as printable or injectable bone filling material silk fibroin and bioactive glass hybrid 3D printed composite scaffold for bone tissue engineering and silk fibroin and I 3K peptide nanofiber scaffold 3D printed cell scaffold. SF can also be blended or combined with other polymers such as alginate, chitosan, and gelatin to alter the rheological properties of the bioink and significantly improve its printability, gelation rate, and cell growth, as well as enhancing its mechanical properties and reducing the degradation rate. When the silk fiber is degummed in boiling or alkaline water, the regenerated silk fibroin, with or without chemical modification, can be made into a starting material for the development of 3D bio-printing ink. Due to its excellent mechanical properties and biocompatibility, with no immunogenicity or toxic side effects, SF fiber has received attention recently in research on the application and development of biomaterials, for example, dye adsorption material, antibacterial material, conductive material, and especially materials that are suitable for medical tissue engineering, drug-carrying composite materials, and bone tissue engineering scaffold.
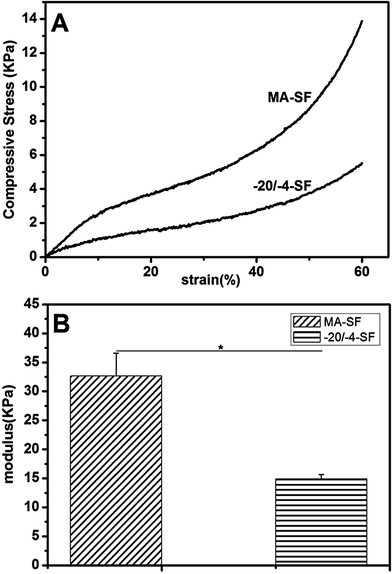
These motifs constitute the crystallinity domain of SF, while other amino acids with larger side chains constitute the amorphous domain. The heavy chain has a GAGAGS repeat sequence accounting for ~70–80% of the total. The silk fibroin has a molecular mass of more than 410 kDa and consists of a heavy chain (~390 kDa) and light chain (~25 kDa). Silk derived from the silkworm Bombyx mori is a natural protein-based polymer composed of approximately 70–80% silk fibroin (SF) and ~20–30% silk sericin. In addition, biomaterials used as bioink should be inexpensive, easy to obtain, and simple to manufacture. When viscosity is high, a greater extrusion force is needed during the 3D printing process of bioink, which could block the nozzle, as well as damage cell vitality. The swelling rate affects the formation of the two-dimensional morphology of the bioink after extrusion, and the appropriate swelling rate will improve the resolution of bioprinting products. The surface tension of bioink has a strong influence on cell attachment, distribution, and development in 3D structures. During the process of 3D printing, the printability of bioink mainly depends on its surface tension, swelling rate, and viscosity. To function as a biological ink, biological material must be cell-friendly, cell-compatible, have strong mechanical properties, and be able to adhere to cells under physiological conditions. The techniques described here provide potential new applications for the recycling and utilization of sericin, which is a waste product of silk processing. Thus, the SP bioinks obtained could be used to print different types of SP composite scaffolds adapted to a variety of applications, including cells, drugs, tissues, etc. The L929 cells adhered, stretched, and proliferated well on the SP composite scaffold. The bovine insulin release rates reached 61% and 56% after 5 days. The swelling properties and resistance to protease hydrolysis of the SP scaffolds containing sericin were improved. The thermal decomposition temperatures of the SF/WS (10%) and SF/ILS (20%) scaffolds, mainly composed of a β-sheet structures, were 3 ☌ and 2 ☌ higher than that of the SF scaffold alone, respectively. The compressive strength of the SF/ILS (20%) scaffold added to G-ILS was 45% higher than that of the SF scaffold alone. After adding I2959 (a photoinitiator), the SP bioinks were prepared with phosphate buffer (PBS) and subsequently bioprinted into various SP scaffolds with a 3D network structure. When the three silk proteins (SPs) were individually grafted with glycidyl methacrylate (GMA), three grafted silk proteins (G-SF, G-WS, G-ILS) were obtained.
The inner layers of sericin (ILS) were also prepared from the degummed silk in boiling water by 120 ☌ water treatment. The whole sericin (WS) can not only be recycled, but completely degummed silk fibroin (SF) is also obtained in this process. This paper describes the use of silk protein, including fibroin and sericin, from an alkaline solution of Ca(OH) 2 for the clean degumming of silk, which is neutralized by sulfuric acid to create calcium salt precipitation.
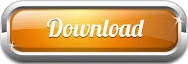